Document details
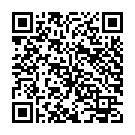
Abstract
Implementing space debris mitigation concepts is a prerequisite to any new spacecraft design and future missions. Beside the several strategies to remove, decrease and control the amount of space debris, protection and shielding from hypervelocity impacts are needed. In the last two decades, self-healing materials have become increasingly interesting due to their capability to recover their integrity and functionality after damage. A particular class of thermoplastic polymers called ionomers have clearly demonstrated their self-repair ability and efficiency after high velocity ballistic impact.
In this work, Ethylene-Methacrylic Acid (EMAA) based ionomer has been investigated due to its excellent damage recovery following high velocity impacts. Numerous experimental data were collected in the years ranging from physical and mechanical experiments to hypervelocity impact tests to better understand and characterize this material and its potential application to space.
The first outcome of this research is related to the ballistic puncture tests used to assess EMAA self-healing performance under different conditions as sample thickness and bullet speed, diameter and shape. Bullet speeds from low velocity to hypervelocity (up to 4 km/s) were considered, and the experiments led to the definition of a critical ratio between sample thickness and bullet diameter below which full repair does not occur. Previous studies also showed that these parameters significantly influence yield strength and post-yield behaviour, and detected a temperature increase in the visco-plastic deformation region during high strain rate tests. This proved to influence the self-repairing mechanism during high-energy impact tests. The obtained results suggest that EMAA has a real potential for applications in complex structures to repair high velocity impact damages. For example, complete healing was observed after impacting 2 mm, 3 mm and 5 mm thick EMAA samples with 1.5 mm diameter projectiles traveling at 1.93 km/s, 1.8 km/s and 4.1 km/s, respectively. Furthermore, a peculiar fragmentation behaviour was observed during impact experiments leading to a sensible lower amount of debris generated compared to metals or composites.
Following the experimental campaign, a theoretical model was defined to capture the material behaviour during the self-healing process, and a numerical model was introduced to simulate this process under high energy impact conditions. After determining the values of the material model parameters, numerical results and experimental data were compared. Once the model was defined, high velocity impacts were simulated in a commercial Finite Element analysis (FEA) software through a Smoothed Particle Hydrodynamics (SPH) formulation. In conclusion, it was proven that a numerical model can be used to simulate the self-healing process, even though additional characterization is required to achieve a more accurate model able to simulate the complete self-healing process.
Implementation of self-healing concepts for ballistic impact protection will open new ways to design and protect space structures from space debris. This concept can be extended for protection against any hypervelocity hazards (e.g.: micrometeoroids) in space, on planets or on the Moon and for any parts including inflatable structures.
Preview
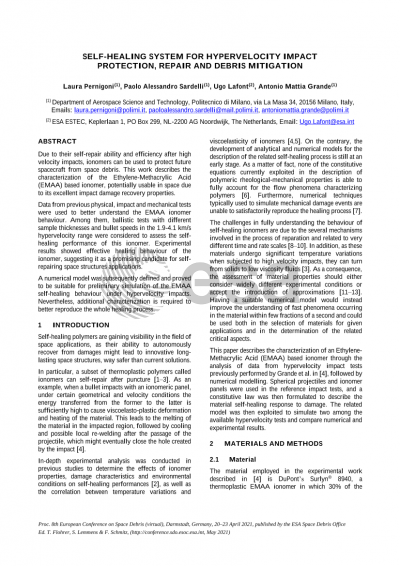
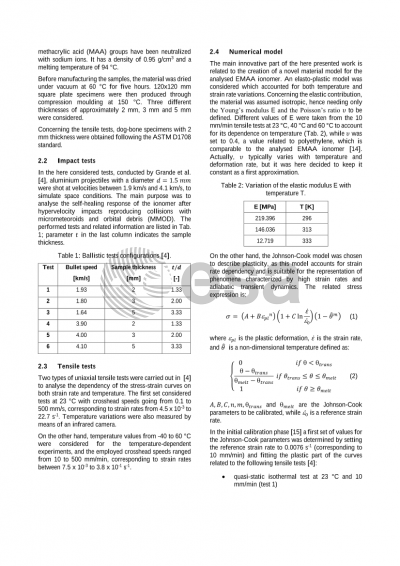
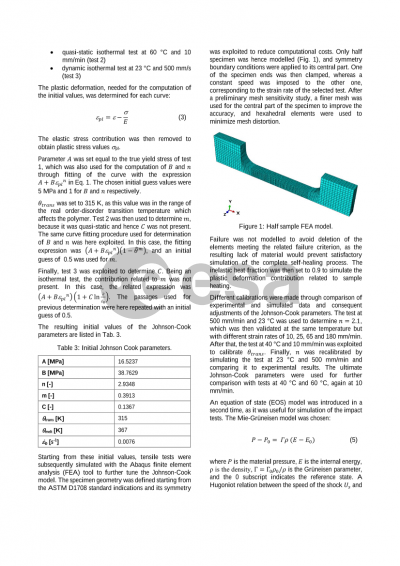
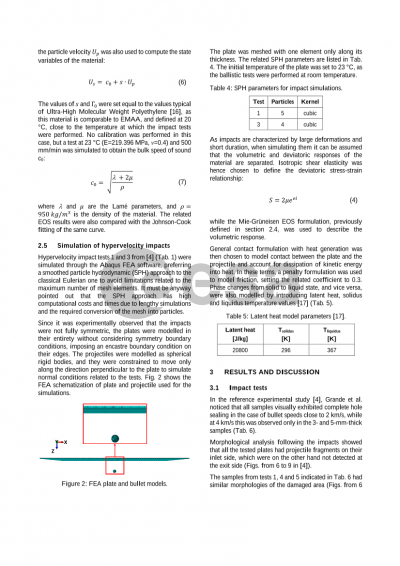
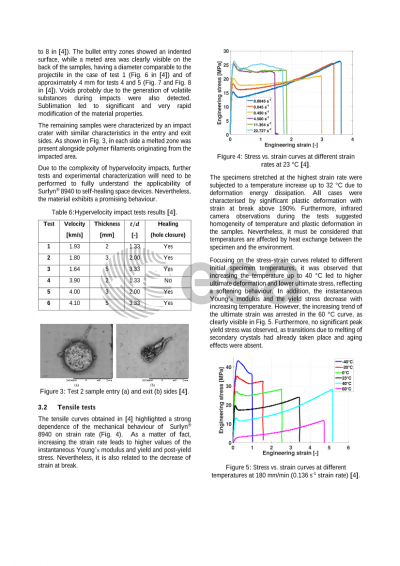
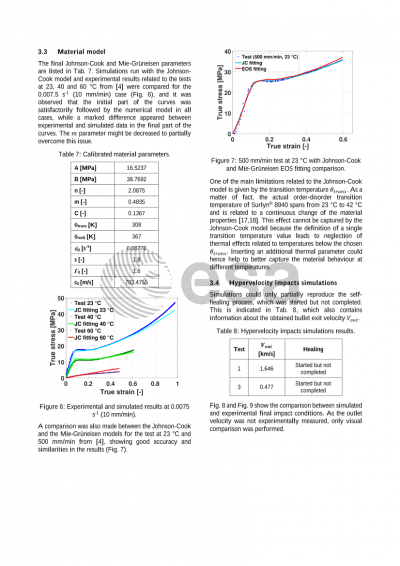
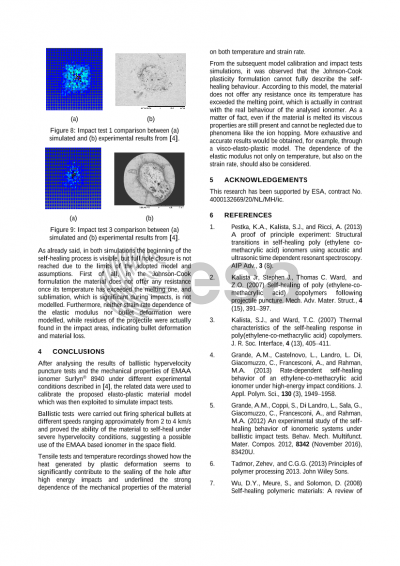
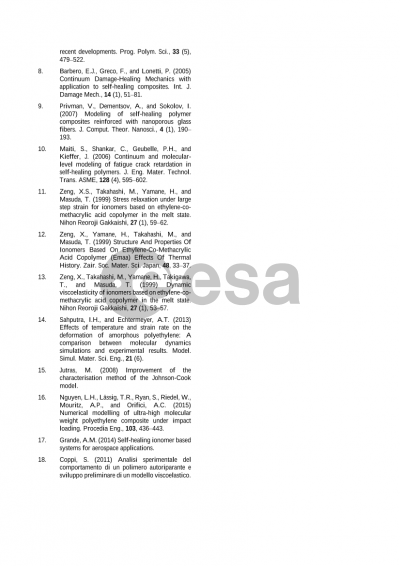