Document details
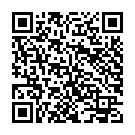
Abstract
With the growing value of the geosynchronous ring for communications and observation, understanding the debris in this orbital environment is all the more important. Debris objects of particular interest are retired geosynchronous satellites. While the orbital evolution of these satellites has been extensively studied, little is understood about their rotational dynamics. However, many are known to spin rapidly or have evolving rotational motion. Better knowledge of this rotational motion stands to improve estimates for attitude dependent solar radiation pressure forces, help assess potential for material shedding, and aid in efforts to mitigate space debris growth through on-orbit component recycling. Therefore, our aim is to better understand and characterize the rotational motion of retired geosynchronous satellites through ground-based optical observation.
Here we present our reduced CCD photometry of several retired geosynchronous satellites and a preliminary analysis of the resulting light-curves to extract rotation periods. These data were collected at Lowell Observatory in Flagstaff, Arizona from June –July 2016 using the 1.8 m Perkins and 1.1 m Hall optical telescopes. A parallel goal of this campaign was to assess the viability of large equatorial mount, astronomical telescopes for high slew-rate satellite observations. At end of life, geosynchronous satellites are generally boosted above the main ring and decommissioned, where they drift westward at 3-5 degrees per day. This slow motion means particular satellites are continuously visible for several weeks and return to view every 2-3 months. As satellites of interest came into view, they were tracked and observed for different time spans, depending on how rapidly the image to image satellite photon count varied. Large image to image variation suggested a fast spin rate and shorter observation arc required to extract the rotation periods. The exposure time for all images was 1 second, with a sampling interval of 5-6 seconds. The images were gathered using either a VR or R filter, with all images in an observation arc having the same filter. The images were then reduced using the Image Reduction and Analysis Facility (IRAF) software package to determine each satellite’s magnitude variation as a function of time.
These resulting light-curves were then analyzed to extract rotation periods. For clearly periodic light-curves, period folding or a low order Fourier series fit can be used to determine each satellite’s rotation period. A light-curve with no obvious repetition suggests that the satellite is in a tumbling rotation state. In this case analysis becomes more involved because there are two fundamental rotation periods for tumbling motion. One period corresponds to rotation around either of the satellite’s extremal axes and the second period to precession and nutation of that axis about the rotational angular momentum vector. To extract tumbling rotation periods, Fourier transforms or a two-dimensional Fourier series fit can be used. To help narrow down and validate candidate period pairs obtained from these two methods, one can leverage constraints derived from torque-free rigid body dynamics theory. For a satellite with particular moments of inertia, there are some period combinations that are physically impossible. Since the mass properties of many of our observed satellites are known or can be estimated, these dynamical constraints can help to select possible period pairs. Preliminary light-curve analysis suggests that at least one retired satellite has evolved from uniform rotation to tumbling motion since previous observations in mid-2014.
Preview
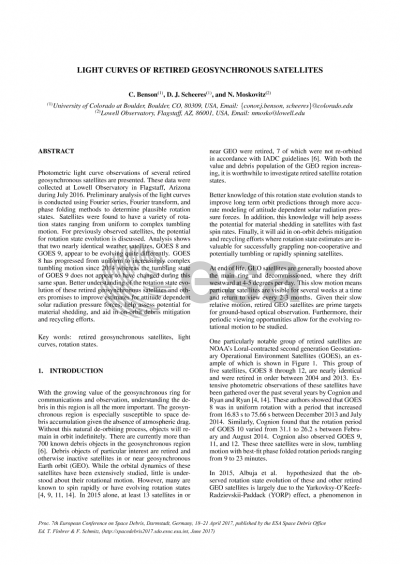
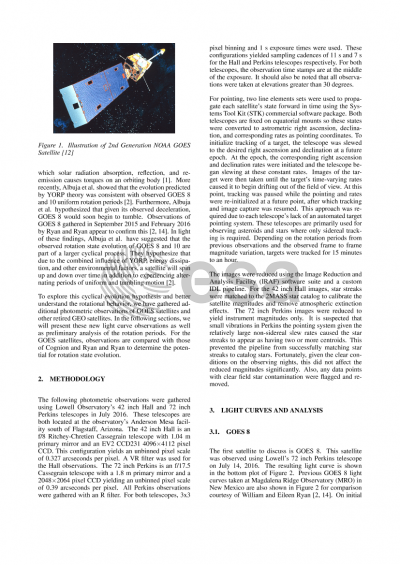
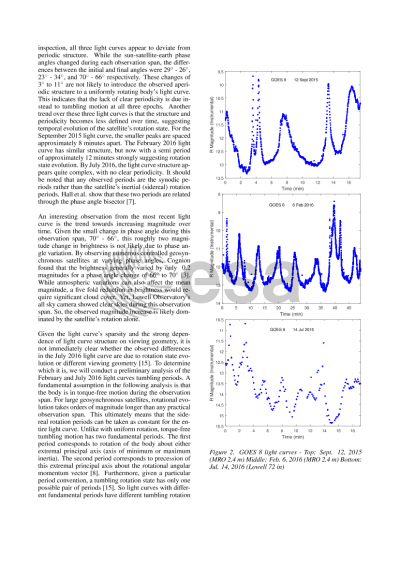
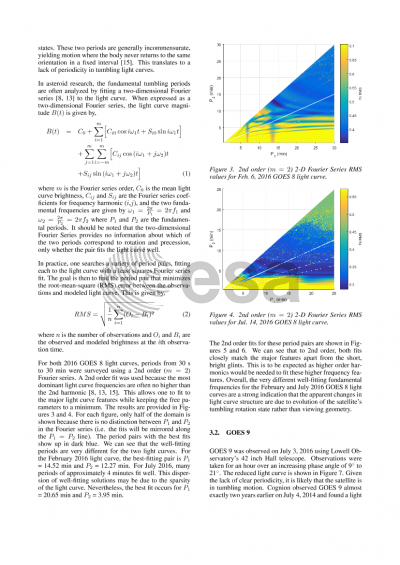
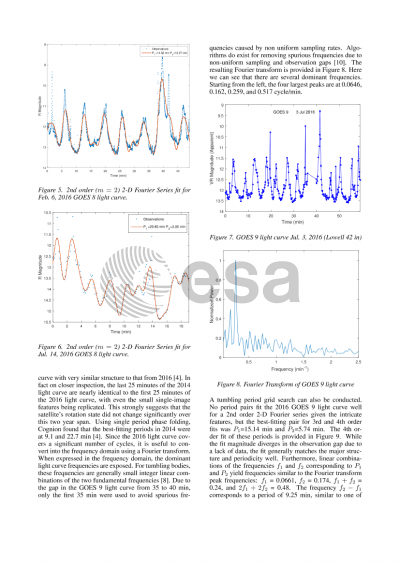
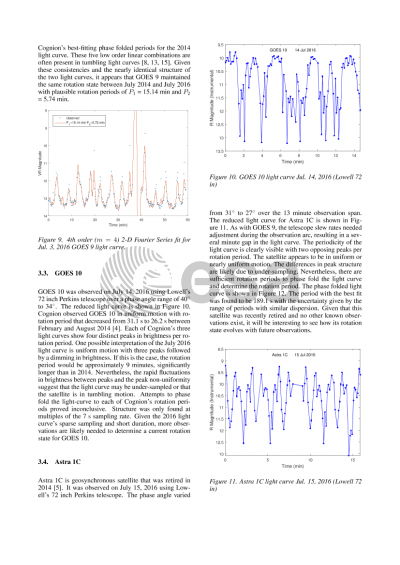
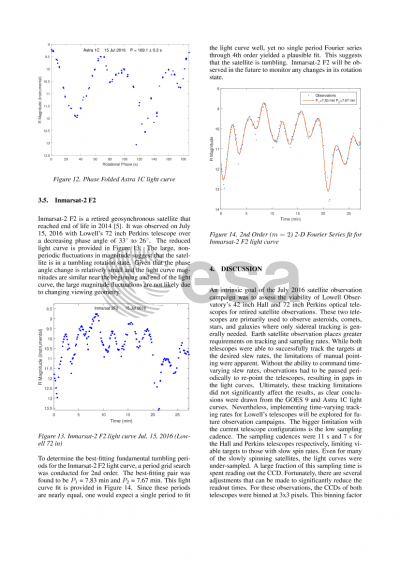
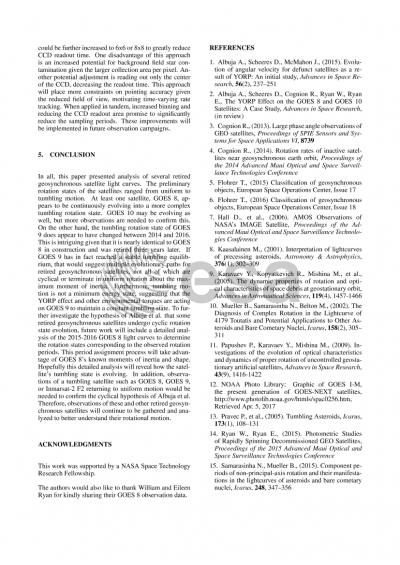